In this blog I will try to clarify what are the main differences between resting heart rate and heart rate variability (HRV), and why we consider HRV a marker of parasympathetic activity and therefore of your stress response when measured under certain conditions.
Context
Both heart rate and HRV can be used for different applications and measured under different conditions. Here, our application of interest is determining chronic physiological stress levels, which derive from combined strong acute stressors (e.g. a hard workout, intercontinental travel) and long-lasting chronic stressors (e.g. work-related worries, etc.).
By measuring the impact of various stressors (e.g. training or lifestyle) on our resting physiology (heart rate and HRV), we can gain awareness and make meaningful adjustments that can lead to better health and performance.
However, this is the case only if we measure our physiology with an accurate tool and at the right time so that it can be interpreted as a proxy for an actual stress response. The relationship between our stress response and HRV does not hold outside of these very specific conditions, such as morning measurements (e.g. taken with HRV4Training via the phone camera or using a chest strap), which are a well-established and reliable way to assess your HRV.
Given this context, we will now look at the relationship between stress, the autonomic nervous system, parasympathetic activity, resting heart rate, and HRV.
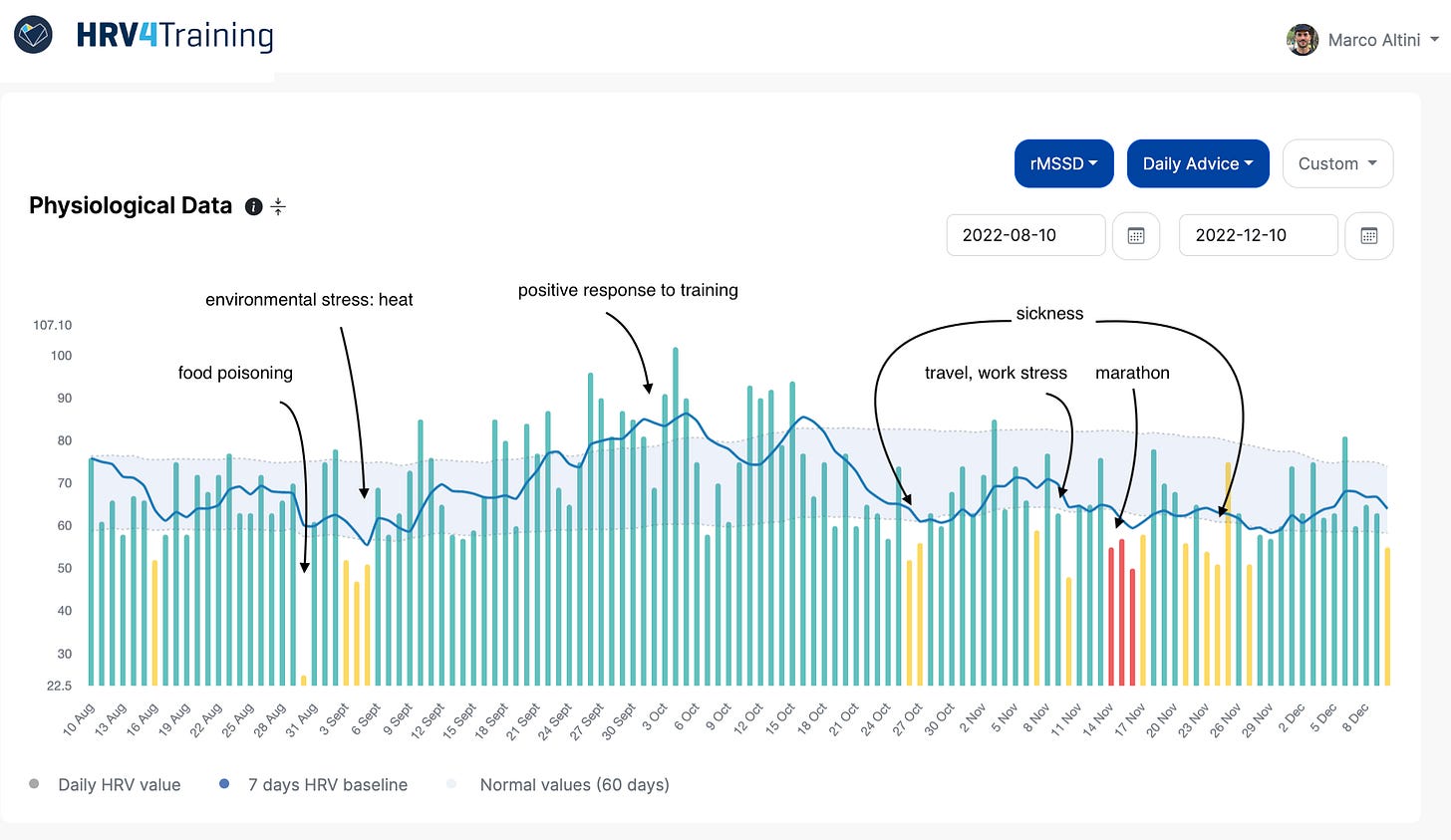
What are we talking about?
Heart rate refers to the number of heart beats over a period of time, typically a minute. On the other hand, HRV is a term that refers to ways to summarize in a number the variability between heartbeats, because even if we count 60 beasts in a minute, they do not happen exactly every second.
Both your heart rate (number of beats) and your HRV (variations in timing between consecutive beats) are modulated by the autonomic nervous system in response to stress. This means that the autonomic nervous system influences heart rhythm.
The autonomic nervous system is the part of your nervous system that controls and regulates many functions in your body, from the heart beating to respiration, ~without conscious control. Thus, heart rate and HRV can indirectly capture changes in autonomic activity non-invasively.
Physiology: bird’s-eye view
How does the heart beat?
The heart has its own pacemaker, meaning that there’s a spot (called sinoatrial node) that generates electric potentials that initiate contractions, resulting in heartbeats. If you had no other mechanism to modulate heart rhythm, your heart would beat at approximately 100 beats per minute (bpm), due to this pacemaker. This intrinsic firing rate is rather constant (hence ~no variability or HRV is present in this state).
On top of this basic mechanism, the heart is innervated by the autonomic nervous system. If you are not new to HRV, you probably heard about the two main branches of the autonomic nervous system: the sympathetic and parasympathetic nervous systems. At this point, you probably heard also that the sympathetic nervous system is responsible for stimulating the body’s fight or flight response (when we need action, resulting in increased heart rate and reduced HRV), while the parasympathetic nervous system is mainly responsible for the body’s resting functions (resulting in reduced heart rate and increased HRV).
This is all true, but we need to remember our context here: measuring physiology at rest. At rest, the body is predominantly parasympathetic. This means that what we measure and quantify is almost entirely parasympathetic activity, more than some sort of balance between the two branches. While this might sound a bit abstract right now, there are practical implications associated with the metrics used and conclusions we can derive, which I will cover in more detail in this blog.
If you have measured your heart rate, you have seen that most likely it is quite a bit lower than the intrinsic firing rate of 100 bpm. In the general population, anything around 60 or 70 is considered normal. If you exercise quite a bit, your heart rate is probably in the high 40s or low 50s, while if you are a pretty decent athlete, it can go down to the low 30s, as there is a strong link between resting heart rate and cardiorespiratory fitness level. This tells us already that at rest, parasympathetic activity is predominant, as heart rate is highly reduced with respect to the intrisinc rate of firing of the sinoatrial node.
But let’s see how we can verify these considerations.
Understanding autonomic control of the heart
How do we know that heart rate (and HRV) are modulated by the autonomic nervous system? And most importantly for applied use of these metrics: how do we know that increased parasympathetic activity reduces heart rate and increases HRV while sympathetic activity has the opposite effect? At rest, how do we establish that parasympathetic control is dominant, while the sympathetic system has little impact?
All of these questions have been answered by pharmacological blockade. What’s pharmacological blockade? Let’s take a step back and cover basic communication first.
In a nutshell, the human body senses stress through its senses and sends information to the brain, which determines how to deal with them. Sources of stress (stressors) are disruptions that trigger certain reactions as the body tries to maintain a state of balance, also called homeostasis, which is key to ensure optimal functioning. So what happens when we face a stressor? Impulses from the brain, starting in the hypothalamus are sent to (among others) the heart, via the autonomic nervous system, and in particular, this communication happens via neurotransmitters.
The main neurotransmitter of the parasympathetic system is acetylcholine, while the sympathetic system relies mostly on norepinephrine. Thus, when acetylcholine is released, it binds to receptors near the sinoatrial node, and slows down heart rate. This process is pretty quick, with latencies in the order of milliseconds, which means that the parasympathetic system can slow down heart rate almost instantaneously, effectively delaying the next heartbeat (and therefore increasing HRV).
Back to pharmacological blockade. Using drugs (in this case atropine), we can make the heart insensitive to acetylcholine, or in other words, we can inhibit the parasympathetic system’s effect on heart rate. What happens when we do so? Heart rate increases, and HRV reduces. In particular, HRV above frequencies of approximately 0.15 Hz is almost absent. In the next section, we’ll dig deeper into why this is the case (it has to do with respiration).
To inhibit sympathetic activity, we can use another drug (propranolol). As expected, when we use this drug, heart rate decreases. However, the decrease in heart rate is very small, effectively highlighting how at rest, parasympathetic activity is predominant.
Let’s look at the figure below (from this paper, which is only 40 years old):
Here the authors administered the same drugs (propranolol and atropine) in different orders, but the outcome is always the same. When atropine was administered, heart rate jumps up (the parasympathetic system is inhibited), while when propranolol was administered, heart rate slightly reduces. This data, together with results from several other groups, has consistently shown the following:
When we inhibit parasympathetic influence on the heart, heart rate increases dramatically, almost reaching the intrinsic firing rate (100 bpm), and HRV at higher frequencies almost disappears.
When we inhibit sympathetic influence on the heart, heart rate decreases, but just a tiny bit, showing how at rest, the heart is mostly under parasympathetic influence.
These experiments highlight clearly how the autonomic nervous system modulates heart rhythm at rest.
A subtle but important difference between autonomic control of heart rate and HRV
In the figure above, we can see how using different drugs we can inhibit parasympathetic or sympathetic control on heart rhythm (or both). The figure shows the change in resting heart rate, but I have also covered how not only heart rate, but also HRV is highly impacted (to a point where we have almost ~no HRV after atropine injection, the drug that “blocks” the parasympathetic system influence on the heart).
However, there is another key point to highlight. Based on the above, we could for example speculate that parasympathetic activity is already entirely reflected in heart rate. This is not the case because the parasympathetic system doesn’t work like a faucet. The parasympathetic system is not on or off, but acetylcholine release and therefore the slowing down of heart rate happens synchronously during respiration. In particular, during expiration vagal activity is higher, leading to a slowing down of heart rate that impacts HRV, but not necessarily average HR.
Below is a classic example, we can see beat-to-beat differences over time (called RR intervals) first during shallow breathing (small beat-to-beat changes), and then during deep breathing (large oscillations).
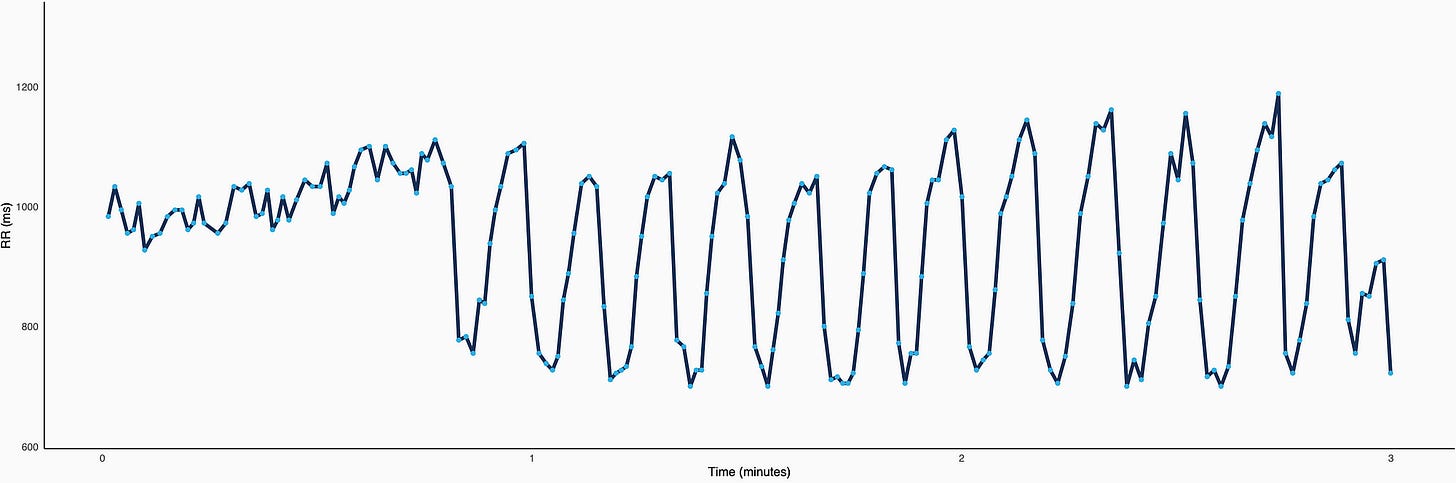
If we compute the average heart rate in the two sections above, so the first ~45" vs the remaining 2'15", we obtain almost the same value (in fact, HR is even a bit lower during shallow breathing, 62 vs 67 bpm). Yet, HRV is much higher during the second part of the recording (doubled, with rMSSD going from 64 to 125 ms).
Let’s recap:
The vagus nerve activity on heart rhythm is quick, and therefore captured by high frequency HRV changes. In a matter of milliseconds, heart rate is slowed down. On the contrary, it takes several seconds for sympathetic activity to impact heart rhythm.
Vagal activity depends on breathing, with increased firing during expiration, and ~no firing during inspiration. This firing pattern originates in the brain and causes increased HRV, but are not captured by heart rate alone. This is a key peculiarity of HRV as low modulation of heart rhythm during breathing (read: low HRV) is associated with a number of adverse outcomes.
While beat-to-beat changes are exaggerated by deep breathing in the figure above, beat-to-beat variability at rest is always influenced by parasympathetic activity during each breathing cycle. Thus, HRV is more sensitive to parasympathetic activity than heart rate alone.
While the theory covered here is key to understanding why certain processes are typically better captured by HRV, you don’t have to take my word for it, we already have massive amounts of data backing this up. This is the beauty of our times.
Parasympathetic activity and HRV indices
So far I have talked about HRV in broader terms, without getting into the specific of the different ways it can be quantified (called features or indeces).
It follows from what I have described above, that if we want to quantify parasympathetic activity, and therefore capture high-frequency changes in beat-to-beat differences, we should use mathematical methods able to capture such changes. The most commonly used HRV feature is called rMSSD and is what you can find in HRV4Training, Oura, Whoop, etc. — because it is a simple mathematical way to quantify fast beat-to-beat changes. Similarly, the high-frequency power (HF) captures the ~same mechanisms.
Personally, I consider rMSSD a better choice for various reasons (less dependent on the exact breathing frequency, more reliable across applications, and a few other reasons that you can find here, should you be interested). The key point here is that common HRV features such as rMSSD and HF capture mathematically the fast changing nature of parasympathetic activity, which means that a reduction in these features is typically associated with higher stress.
Takeaways
Let’s try to recap the whole physiological mechanism in a few sentences: as the body tries to maintain a state of balance so that it can function optimally, heart rhythm is influenced by a series of processes going from the brain to the heart via the autonomic nervous system. These processes reflect the level of stress on the body. In particular, we have seen how parasympathetic influence on heart rhythm via acetylcholine release causes heart rate to reduce. Additionally, acetylcholine release has an almost immediate effect on heart rate, delaying instantaneously the following heartbeat. This instantaneous effect, combined with increased parasympathetic activity during the exhale, makes it so that HRV, more than heart rate, can capture resting parasympathetic activity, and thus physiological stress.
Many studies have used pharmacological methods to clearly highlight these pathways and how they impact measurements of heart rate and HRV, for example by blocking acetylcholine receptors (or parasympathetic activity) and quantifying the resulting drop in HRV and increase in heart rate. While there is an obvious relationship between heart rate and HRV, the exact same heart rate can result in dramatically different HRV values. Heart rate does not say anything about beat-to-beat variation (by definition, it is an average) and therefore fails to capture the dynamics of the (fast-acting) parasympathetic system. This does not mean that heart rate is less relevant, but it is simply different, and impacted by other processes.
To conclude:
Heart rhythm (both heart rate and HRV) is influenced by the autonomic nervous system in response to stress.
At rest, parasympathetic activity is predominant, which results in lowered heart rate and increased HRV, with respect to the heart’s intrinsic firing rate.
Due to the timing of parasympathetic activity (which is fast and coupled to breathing), HRV analysis captures information not present in average heart rate alone, highlighting an important difference at the physiological level.
Keep in mind that the link between parasympathetic activity and HRV is valid only under certain conditions (e.g. morning measurements or night data), and is not meaningful when looking at HRV data continuously, due to a myriad of artifacts and other physiological processes that impact HRV data regardless of stress (see this blog for more details).
I hope this was informative, and thank you for reading!
If you’ve enjoyed this post and would like to get more practical on tools and methods to effectively use HRV, check out my guide, below:
How to Show Your Support
No paywalls here. All my content is and will remain free.
As a HRV4Training user, the best way to help is to sign up for HRV4Training Pro.
Thank you for supporting my work.
Endurance Coaching
If you are interested in working with me, please learn more here, and fill in the athlete intake form, here.
Marco holds a PhD cum laude in applied machine learning, a M.Sc. cum laude in computer science engineering, and a M.Sc. cum laude in human movement sciences and high-performance coaching.
He has published more than 50 papers and patents at the intersection between physiology, health, technology, and human performance.
He is co-founder of HRV4Training, advisor at Oura, guest lecturer at VU Amsterdam, and editor for IEEE Pervasive Computing Magazine. He loves running.
Social:
When we do morning hrv I believe you tell us to breath normally however it appears that just by concentrating on exhale we can increase our hrv. Why shouldn’t we do that?